What is Cell Imbalance?
In a battery pack made up of multiple cells connected in series, cell imbalance occurs when individual cells have different voltages, capacities, or states of charge (SOC).
This mismatch is common, even with initially identical cells, due to manufacturing variations and uneven usage patterns.
The Culprits: What Causes Cell Imbalance?
Several factors can contribute to cell imbalance:
Manufacturing Imperfections: Despite state-of-the-art production processes, no two cells are perfectly identical. Minute variations in material composition, electrode geometry, or cell assembly can lead to slight performance differences.
Temperature Gradients: Batteries function optimally within a specific temperature range. Uneven heat distribution across a pack, often caused by cooling system limitations or high charge/discharge rates, can exacerbate cell-to-cell inconsistencies.
Ageing and Degradation: All cells age. However, slight variations in cycling history, operating conditions, or internal defects can cause some cells to age faster than others, leading to a progressive loss of capacity and increased impedance
Anatomy of Imbalance: Understanding the Different Types
Cell imbalance manifests in several forms, each with its own contributing factors and detrimental effects:
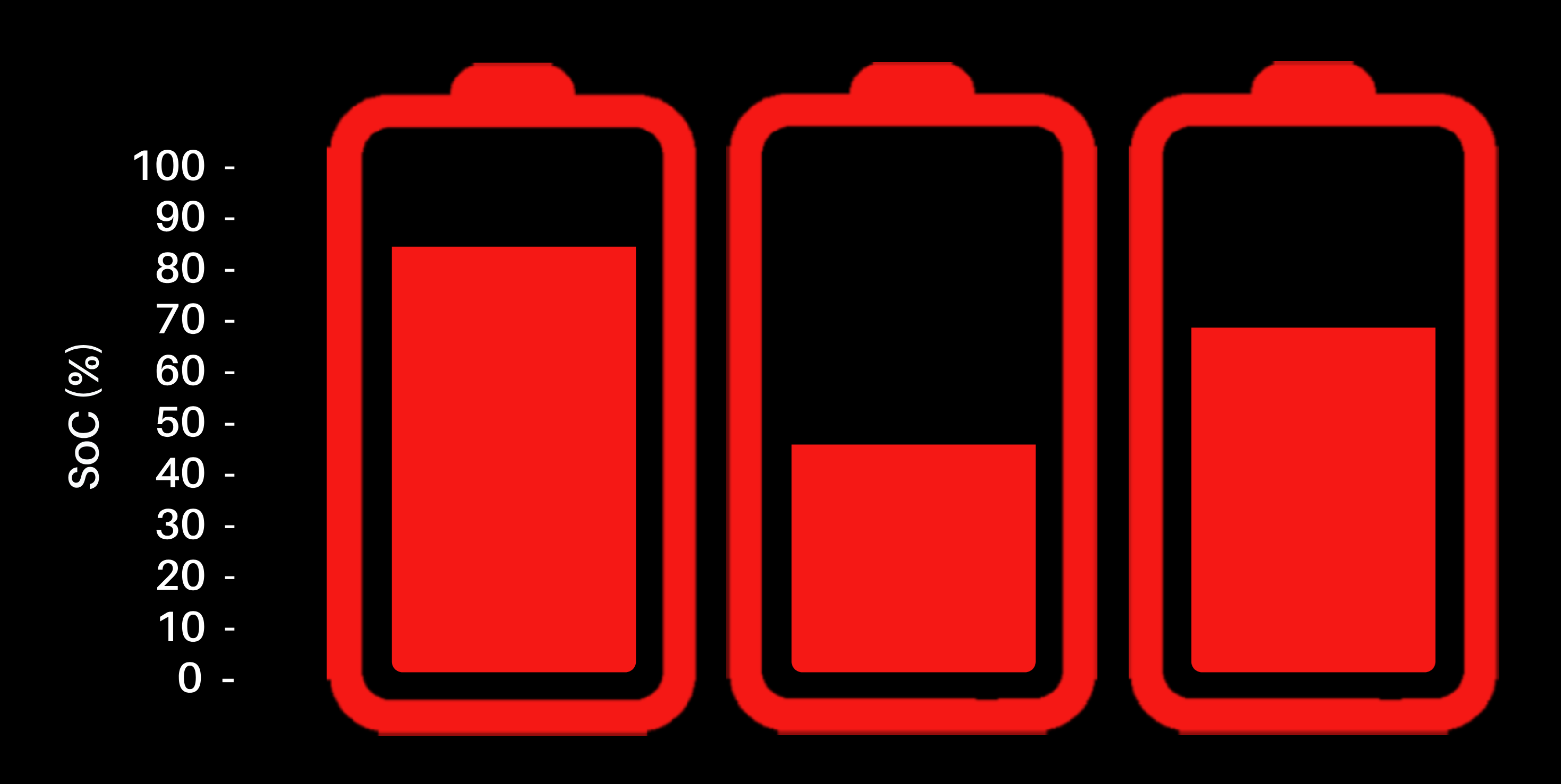
Cell Imbalance
State-of-Charge (SoC) Imbalance: Cells exhibit varying amounts of remaining charge. This is commonly caused by inconsistencies in self-discharge rates, minor temperature variations, and uneven charge/discharge patterns within the pack.
Source: IEEE Transactions on Smart Grid
Capacity Imbalance: Cells possess differing energy storage capabilities. This stems from inherent manufacturing variations in active material quantity and internal geometry, along with uneven cycling history that accelerates degradation rates in certain cells.
Source: Journal of Power Sources:
Impedance Imbalance: Cells display non-uniform internal resistances. These discrepancies result from cell design, manufacturing tolerances, and the degree of degradation over time. Impedance imbalance can limit current flow within the pack and exacerbate SoC imbalance under dynamic load conditions.
Why Cell Imbalance is a problem: Quantifying the Impact of Cell Imbalance
The adverse consequences of cell imbalance aren't theoretical–they can significantly impact the real-world performance and longevity of your battery pack:
Reduced Usable Capacity: Even a small cell imbalance can severely curtail the pack's usable energy. A study published in Batteries Journal illustrates that an SoC imbalance of merely 10% can decrease the effective capacity by up to 20%.
Source: MDPI Journal – Batteries:
Accelerated Degradation: Imbalanced cells are subjected to disproportionate stress. Overcharged cells undergo a rapid decline in capacity due to irreversible lithium plating and side reactions, while chronically undercharged cells risk harmful metallic deposits and voltage reversal. This vicious cycle propagates the imbalance, exacerbating the damage even further.
Source : National Renewable Energy Laboratory (NREL):
Decreased Pack Lifespan: The lifespan of a battery pack is inherently tied to its weakest cell. Persistent imbalance prematurely retires healthy cells alongside their distressed counterparts. Research indicates that a capacity imbalance exceeding 5% can slash a battery's lifespan by 30% or more.
Source : World Electric Vehicle Journal:
Safety Hazards: Extreme cases of cell imbalance push cells beyond their safe operating limits. Severely overcharged or over discharged cells are susceptible to thermal runaway, internal short circuits, and catastrophic events like fires or explosions.
The Art of Balancing: Passive vs. Active Approaches
Two main balancing strategies are used by modern BMS technologies:
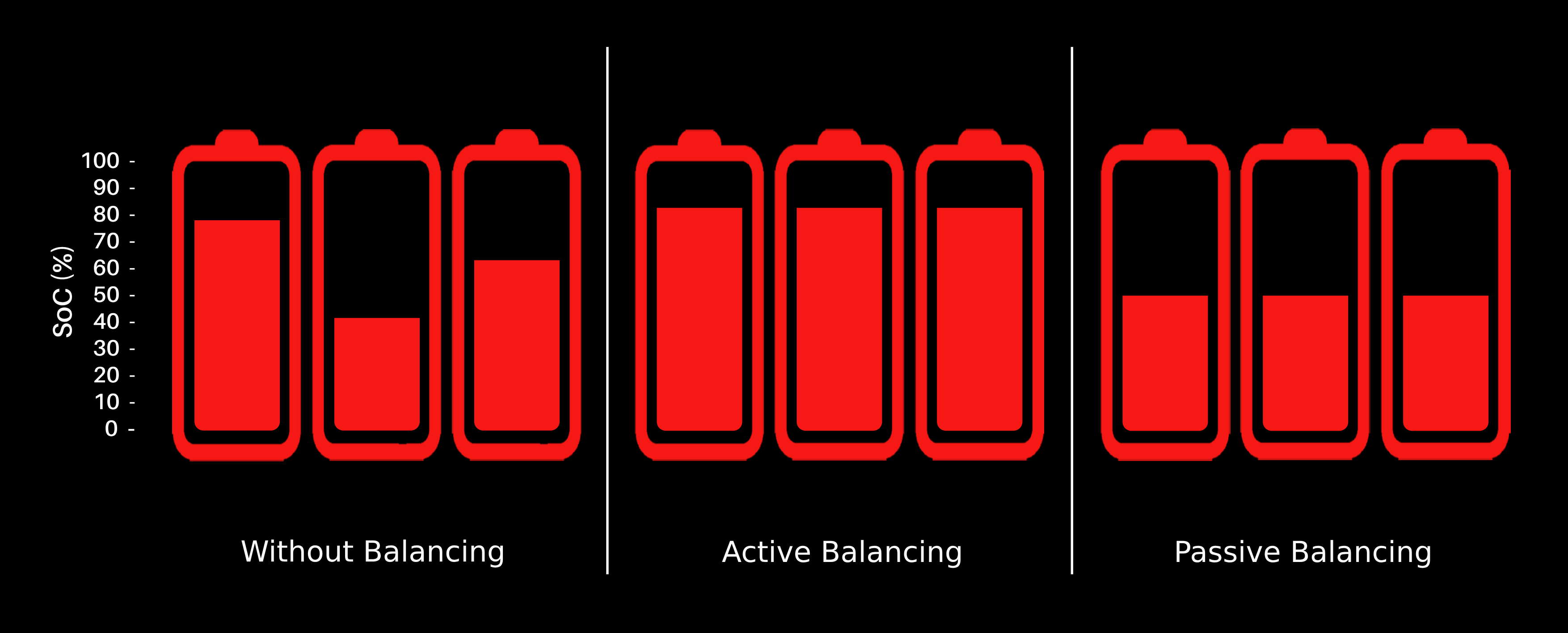
Passive Balancing: This method involves intentionally dissipating excess energy from higher-voltage cells using resistive elements (often called bleed resistors). While renowned for its simplicity and robustness, passive balancing suffers from inherent inefficiency and heat generation.
Source: IEEE Transactions on Vehicular Technology:
Active Balancing: This approach seeks to redistribute energy from stronger cells to weaker ones without intentional wastage. Various topologies exist, utilising capacitors, inductors, or transformers as charge shuttling mechanisms. Despite added complexity, active balancing offers improved efficiency and greater potential for fine-grained control.
Source: Energies Journal:
Choosing Wisely: When to Use Passive vs. Active Balancing
Selecting the right balancing strategy involves a careful analysis of your specific battery pack, its intended application, and budgetary considerations. Here's a breakdown of factors influencing this decision:
Type and Severity of Imbalance: If dealing primarily with minor state-of-charge (SoC) imbalances, passive balancing may be sufficient. However, for significant capacity mismatches and in cases where energy efficiency is paramount, active balancing holds a clear advantage.
Balancing Speed: Passive balancing is a relatively slow process. If frequent, deep charge/discharge cycles are the norm for your battery, the swift rebalancing capabilities of active methods will be vital for long-term pack health.
Thermal Management: Passive balancing dissipates excess energy as heat. If your battery pack is already in a thermally challenging environment, active balancing, with its reduced heat generation, might be a smarter choice
Cost Sensitivity: Passive balancing systems are generally more cost-effective due to their simpler circuitry. Active systems, despite their efficiency benefits, inherently carry additional costs due to switching components and more sophisticated control requirements.
Space Constraints: In applications where space is at a premium (think drones or small form-factor devices), the minimalist footprint of passive balancing could be a compelling factor.
NMC vs LFP: The LFP Balancing Challenge: Why It's Trickier
Lithium-iron-phosphate (LFP) batteries have gained prominence due to their affordability, intrinsic safety characteristics, and long cycle life. However, they introduce complexities for traditional battery management systems, especially regarding cell balancing. Here's why:
Flat Voltage Profile: Unlike NMC (nickel-manganese-cobalt) cells, which exhibit a more linear change in voltage across their state-of-charge (SoC) range, LFP cells feature an exceedingly flat voltage curve throughout most of their usable capacity. This makes relying on voltage as a proxy for SoC remarkably unreliable.
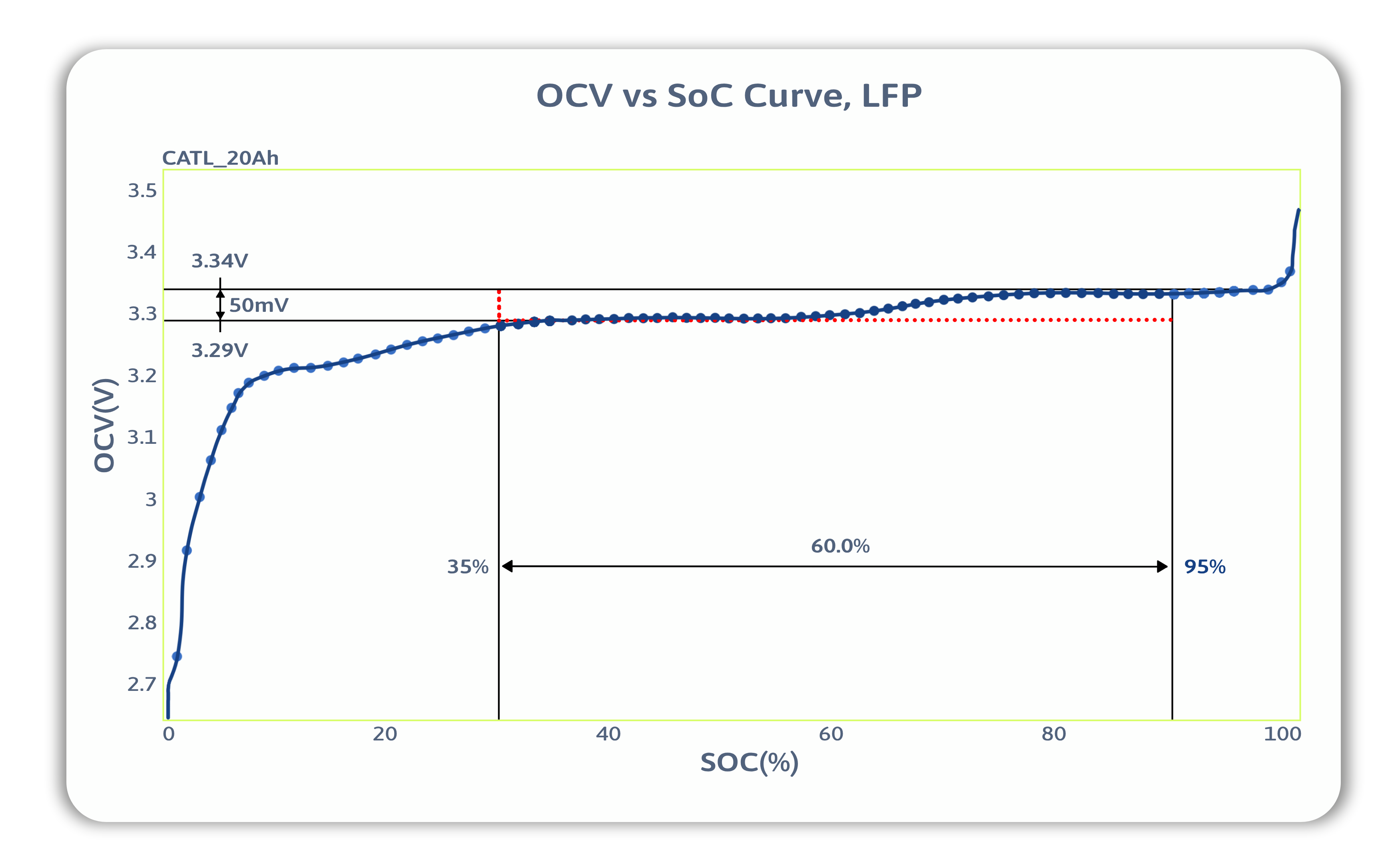
OCV vs SOC Curve For LFP- 50mv Difference can have Significant SoC Change
Vulnerability to Imbalances: The very flatness that gives LFP cells stability during normal operation also leaves them more susceptible to imbalance-related damage. Minor voltage differences are less likely to auto-correct through natural charge redistribution. These imbalances tend to propagate more readily.
Hysteresis Effect: LFP cells demonstrate a more significant hysteresis effect – voltage response is influenced by recent charge/discharge history. This renders even accurate, voltage-based SoC estimation less helpful as it may not match the cell's 'true' internal charge state.
Solving LFP Cell Imbalance: The Power of AI and Physics-Based Modelling
In the context of LFP chemistries, our physics-based model offers a powerful solution for addressing cell imbalance. By incorporating temperature-dependent Single Particle Models (SPMs) that cover a wide frequency range, this model provides a comprehensive understanding of diffusion and double layer capacitance effects. Its linear and integer-order nature, combined with computational efficiency, makes it particularly suitable for real-time applications, such as cell balancing in BMS. Leverageing this physics-based model allows for precise control and estimation functions, enabling effective solutions to cell imbalance challenges in LFP chemistries.Source
Prevent Cell Imbalance: Optimise Battery Packs with LIME AI's Advanced Cell Sorting and Grouping
Cell sorting and grouping: Our sophisticated algorithm is designed to empower the Battery OEMs with an unparalleled tool to combat Cell Imbalance effectively. By leverageing state-of-the-art technology, our algorithm drastically reduces instances of Cell Imbalance, thereby enhancing the overall efficiency and lifespan of battery packs.
LIME AI : Battery Management System
Intelligent, Predictive Algorithms: Our BMS stands at the forefront of LFP battery management. It combines on-board intelligence with cloud-based analytics, harnessing cutting-edge neural networks and machine learning algorithms to accurately predict and address cell imbalances. This unique approach safeguards battery health, maximises performance, and ensures exceptional reliability for your LFP-powered applications.
Conclusion
" Cell imbalance can no longer afford to be an afterthought. By using advanced BMS like LIME BMS which has synergy between Cloud and Edge, we unlock our batteries' full potential. And remember, while every application possesses unique requirements, the goal remains constant: maintaining balance, promoting longevity, and championing safety as we journey towards a sustainable energy future. "