Introduction
Lithium-ion (Li-ion) batteries are the powerhouse behind our modern devices, from smartphones and laptops to electric vehicles (EVs). However, like any technology, they are not immune to degradation over time. Understanding the mechanisms of degradation, their effects on battery performance, and solutions to mitigate them is crucial to maximize the lifespan and utility of Li-ion batteries.
Causes of Lithium-Ion Battery Degradation
Li-ion battery degradation isn't caused by a single factor but rather by a complex interplay of causes. Here's a breakdown of the primary contributors:
Calendar Ageing: Even when unused, Li-ion batteries age due to continuous side reactions occurring within the cell. Temperature plays a significant role, with higher temperatures accelerating these side reactions.
Cycle Ageing: The repeated charging and discharging of Li-ion batteries causes structural changes within the electrodes. Lithium ions lose mobility over time, and the electrode materials themselves can crack and degrade, leading to a loss of capacity and power.
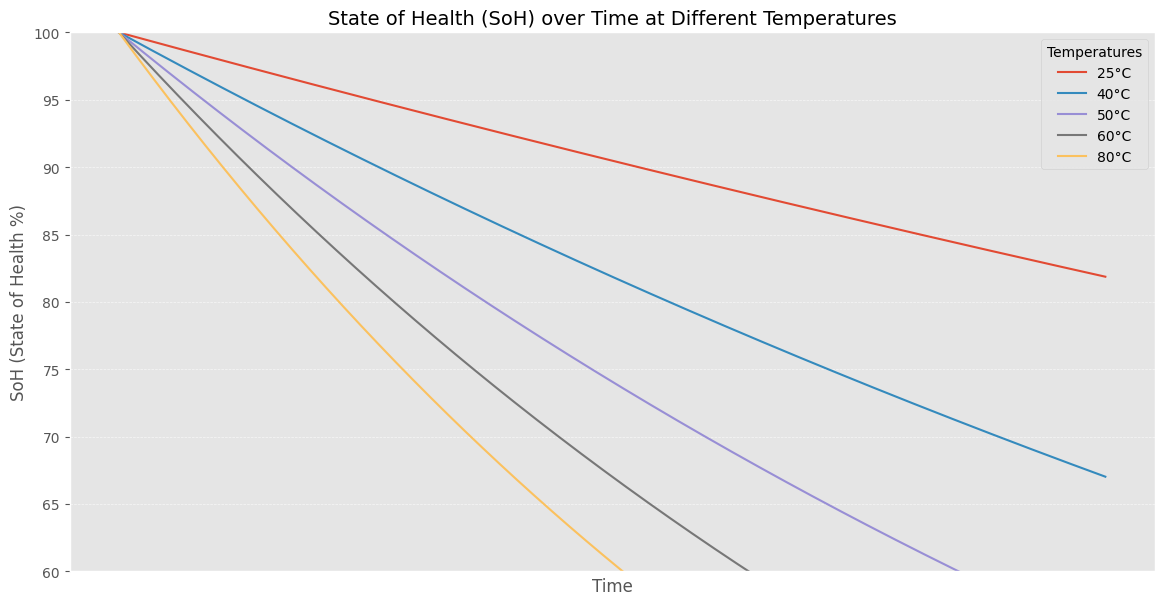
State of Health (SoH) Across Time Under Varied Temperature Conditions.
High Temperatures: A multi-faced threat extreme heat exacerbates virtually all degradation mechanisms. Elevated temperatures promote side reactions within the battery, including:
- SEI Formation and Growth: During the first few charging cycles of a lithium-ion battery, a thin film forms on the surface of the anode through electrolyte decomposition. This is called the Solid-Electrolyte Interphase (SEI). A stable SEI layer is actually beneficial – it prevents further electrolyte breakdown, protecting the anode.
- However, the SEI layer can grow thicker over time, especially in conditions like high
temperatures or overcharging. A thick SEI layer hinders the movement of lithium ions, increasing
internal resistance and reducing battery capacity.
Source: SEI Formation and Growth - Royal Society of Chemistry
- Electrolyte Decomposition: High temperatures can accelerate the breakdown of the electrolyte, generating unwanted by-products that further degrade battery performance.
- Structural Damage: Prolonged exposure to heat can cause warping, cracking, or other physical damage to the electrodes and separator components within the battery.
Overcharging and Deep Discharging: Stressing the battery by routinely charging it to 100% or discharging it fully places it outside its ideal operating window. Overcharging can lead to lithium plating on the anode, while deep discharging can cause irreversible damage to the cathode.
- Lithium Plating: Overcharging, especially at low temperatures, can cause lithium ions to deposit as metallic lithium on the anode surface, rather than inserting into the electrode structure. This lithium plating reduces active lithium available for capacity and can lead to dendrite formation, posing a safety risk.
- Cathode Degradation: Deep discharging can irreversibly damage the structure of the cathode, reducing its ability to store and release lithium ions.
Fast Charging: Though convenient, fast charging stresses the battery because of increased
heat generation and accelerated structural changes in the electrodes, contributing to faster
degradation.
Source: Cause of
Battery Degradation - Exro Technologies
Effects of Lithium-Ion Battery Degradation
The most obvious effects of Li-ion battery degradation are:
Capacity Loss: Over time, the battery's ability to hold a charge decreases, leading to shorter runtimes for devices or reduced range for EVs.
Increased Internal Resistance: Degradation leads to higher internal resistance within the battery, making it less efficient. This means the battery generates more heat during use, further accelerating degradation processes.
Safety Risks: Extreme degradation can, in rare cases, cause thermal runaway. This is when the battery overheats uncontrollably, potentially leading to fires or explosions.
Solutions and Mitigation Strategies
While degradation is inevitable, several strategies can help slow down the process and maximize the lifespan of Li-ion batteries:
Temperature Management: Keep batteries within their optimal operating temperature range (usually between 15°C and 35°C). Avoid exposing batteries to extreme heat or cold, especially during operation or charging.
Partial Charging and Discharging: Avoid full charge (100%) or deep discharge cycles whenever possible. Aim to maintain a State of Charge (SoC) between 20% to 80% for most applications.
Moderate Charging Rates: Opt for slower charging rates when feasible to reduce internal stress on the battery.
Proper Storage: If storing a Li-ion battery for an extended period, partially charge it (around 40% to 50%) and store it in a cool, dry place.
The Essential Role of a Battery Management System (BMS):A sophisticated BMS is one of the most powerful tools for combating battery degradation. It intelligently monitors and regulates a battery's health throughout its life cycle. Here's how a BMS helps:
- Optimized Charging and Discharging: A BMS actively controls charging and discharging processes to avoid overcharging, deep discharging, and excessive currents. This helps prevent dendrite formation and minimizes structural stress on the electrodes.
- Thermal Management: A BMS may integrate with cooling or heating systems to keep the battery within its optimal temperature range. It can warn users of overheating conditions and adapt charging protocols accordingly.
- Cell Balancing: In multi-cell battery packs, a BMS ensures even charge distribution and prevents individual cells from being overstressed, improving overall longevity.
Source: Mitigating Battery Degradation - • National Institutes of Health (NIH)
Effectiveness of a BMS in Extending Battery Life
A well-designed BMS can significantly extend battery's lifespan. While the exact degree of improvement varies depending on the battery's chemistry and usage patterns, studies suggest the following potential benefits:
Calendar Life Extension: A BMS can reduce side reactions and maintain the battery in a healthier state during storage, potentially slowing calendar ageing.
Cycle Life Increase: By optimizing charging, discharging, and balancing cells, a BMS can significantly increase the number of cycles a battery can endure before reaching capacity fades.
Choosing the Right BMS
Not all BMS systems are equal: To maximize battery life extension, look for a BMS with robust algorithms, precise monitoring capabilities, and features tailored to your specific battery chemistry and application. Consider factors like cell-balancing accuracy, thermal management capabilities, and the sophistication of its health analytics.
The Advantage of Lime BMS: Our BMS is meticulously engineered to optimize battery longevity. Features such as advanced cell balancing algorithms, predictive analytics, or tailored charging profiles can potentially extend battery life by up to 20% compared to traditional BMS designs.
The Power of LIME AI Battery Analytics and SoH Predictions
Traditional battery monitoring often relies on basic indicators like voltage, current, and temperature. However, the evolution of battery analytics and advanced algorithms opens a new frontier in degradation management:
Cloud-Based Analytics: Vast amounts of battery data can now be sent to powerful cloud-based systems. These systems analyse complex patterns and subtle changes that may signal early signs of degradation, undetectable by on-device monitoring alone.
State of Health (SoH): SoH is a measure of a battery's current condition compared to its ideal state. Advanced analytics and machine learning models enable more accurate SoH estimations, going beyond simple capacity measurements.
Predictive Analytics: By identifying specific degradation patterns, algorithms can predict future battery health and estimate when performance might fall below critical thresholds.
Benefits of Battery Analytics in Degradation Management
Tailored Battery Care: Analytics provide insights into how specific usage patterns affect battery health, enabling personalized recommendations for extending lifespan.
Proactive Maintenance: Predictive analytics facilitate early warnings before critical failures, allowing for timely replacement or preventative maintenance interventions.
Improved Battery Design: Large datasets aid battery manufacturers in pinpointing weaknesses and designing future generations of batteries with superior durability.
Enhanced Second-Life Value: Robust SoH assessment supports the growing second-life battery market, especially for EVs, by providing transparency into a used battery's remaining potential.
Innovations for Longer Lasting Batteries
Researchers are actively exploring new materials and technologies to push the boundaries of Li-ion battery longevity:
Improved Electrolyte Formulations: Research into electrolyte additives and new electrolyte combinations aims to enhance stability and reduce side reactions.
Advanced Electrode Materials: Materials like silicon-based anodes or novel cathode chemistries promise to improve cycle life and reduce degradation.
Solid-State Electrolytes: These replace the liquid electrolyte in traditional Li-ion
batteries, potentially opening a path to safer batteries with higher energy density and reduced
degradation.
Source:
Electrolyte Formulations - The Faraday Institution
Table of Contents
- Introduction
- Causes of Lithium-Ion Battery Degradation
- Effects of Lithium-Ion Battery Degradation
- Solutions and Mitigation Strategies
- Effectiveness of a BMS in Extending Battery Life
- Choosing the Right BMS
- The Power of LIME AI Battery Analytics
- Benefits of Battery Analytics in Degradation Management
- Innovations for Longer Lasting Batteries